Is Fe(OH)2 soluble in alkalis?
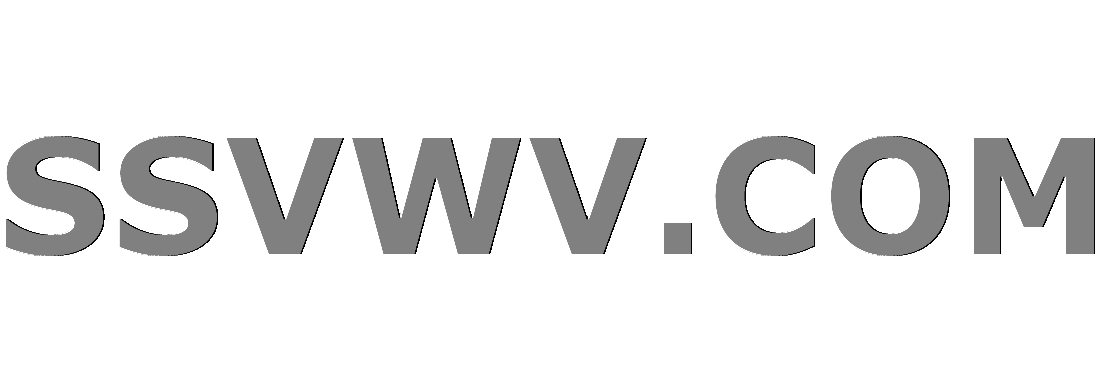
Multi tool use
$begingroup$
I have just started inorganic chemistry in school and I have just learnt about metal aqua ions and how they react in water. I learnt that $ce{[Fe(H2O)6]^2+}$ reacts in water by losing a $ce{H+}$ acting as a Bronsted-Lowry acid producing $ce{H+}$ ions. Due to this reaction we can test for metals such as $ce{Fe^2+}$ by reacting it with $ce{NaOH}$. This is because the $ce{OH-}$ ions react with the $ce{H+}$ ions produced pushing the equilibria to the right forming a insoluble precipitate. My questions is, why does the reaction stop there? The reaction stops once $ce{[Fe(OH)2(H2O)4]}$ forms which is the insoluble precipitate but why doesn't it continue to form $ce{[Fe(H2O)3(OH)3]-}$ and become soluble again?
inorganic-chemistry acid-base aqueous-solution coordination-compounds transition-metals
$endgroup$
add a comment |
$begingroup$
I have just started inorganic chemistry in school and I have just learnt about metal aqua ions and how they react in water. I learnt that $ce{[Fe(H2O)6]^2+}$ reacts in water by losing a $ce{H+}$ acting as a Bronsted-Lowry acid producing $ce{H+}$ ions. Due to this reaction we can test for metals such as $ce{Fe^2+}$ by reacting it with $ce{NaOH}$. This is because the $ce{OH-}$ ions react with the $ce{H+}$ ions produced pushing the equilibria to the right forming a insoluble precipitate. My questions is, why does the reaction stop there? The reaction stops once $ce{[Fe(OH)2(H2O)4]}$ forms which is the insoluble precipitate but why doesn't it continue to form $ce{[Fe(H2O)3(OH)3]-}$ and become soluble again?
inorganic-chemistry acid-base aqueous-solution coordination-compounds transition-metals
$endgroup$
$begingroup$
Actually precipitate isn't [Fe(OH)2(H2O)4]. Precipitation starts with it, but it's rather complex condensation/polymerisation process.
$endgroup$
– Mithoron
9 hours ago
add a comment |
$begingroup$
I have just started inorganic chemistry in school and I have just learnt about metal aqua ions and how they react in water. I learnt that $ce{[Fe(H2O)6]^2+}$ reacts in water by losing a $ce{H+}$ acting as a Bronsted-Lowry acid producing $ce{H+}$ ions. Due to this reaction we can test for metals such as $ce{Fe^2+}$ by reacting it with $ce{NaOH}$. This is because the $ce{OH-}$ ions react with the $ce{H+}$ ions produced pushing the equilibria to the right forming a insoluble precipitate. My questions is, why does the reaction stop there? The reaction stops once $ce{[Fe(OH)2(H2O)4]}$ forms which is the insoluble precipitate but why doesn't it continue to form $ce{[Fe(H2O)3(OH)3]-}$ and become soluble again?
inorganic-chemistry acid-base aqueous-solution coordination-compounds transition-metals
$endgroup$
I have just started inorganic chemistry in school and I have just learnt about metal aqua ions and how they react in water. I learnt that $ce{[Fe(H2O)6]^2+}$ reacts in water by losing a $ce{H+}$ acting as a Bronsted-Lowry acid producing $ce{H+}$ ions. Due to this reaction we can test for metals such as $ce{Fe^2+}$ by reacting it with $ce{NaOH}$. This is because the $ce{OH-}$ ions react with the $ce{H+}$ ions produced pushing the equilibria to the right forming a insoluble precipitate. My questions is, why does the reaction stop there? The reaction stops once $ce{[Fe(OH)2(H2O)4]}$ forms which is the insoluble precipitate but why doesn't it continue to form $ce{[Fe(H2O)3(OH)3]-}$ and become soluble again?
inorganic-chemistry acid-base aqueous-solution coordination-compounds transition-metals
inorganic-chemistry acid-base aqueous-solution coordination-compounds transition-metals
edited 9 hours ago


Mithoron
3,60782844
3,60782844
asked 13 hours ago
JamesJames
276
276
$begingroup$
Actually precipitate isn't [Fe(OH)2(H2O)4]. Precipitation starts with it, but it's rather complex condensation/polymerisation process.
$endgroup$
– Mithoron
9 hours ago
add a comment |
$begingroup$
Actually precipitate isn't [Fe(OH)2(H2O)4]. Precipitation starts with it, but it's rather complex condensation/polymerisation process.
$endgroup$
– Mithoron
9 hours ago
$begingroup$
Actually precipitate isn't [Fe(OH)2(H2O)4]. Precipitation starts with it, but it's rather complex condensation/polymerisation process.
$endgroup$
– Mithoron
9 hours ago
$begingroup$
Actually precipitate isn't [Fe(OH)2(H2O)4]. Precipitation starts with it, but it's rather complex condensation/polymerisation process.
$endgroup$
– Mithoron
9 hours ago
add a comment |
2 Answers
2
active
oldest
votes
$begingroup$
This is a pretty interesting question. First of all, you are right, if you ever happened to see a yellow $ce{Fe^3+}$ solution that was no pure aqua-complex but more likely $ce{[Fe(H2O)5(OH)]^2-}$. The real $ce{[Fe(H2O)6]^3+}$ for example, like it is formed in conc. $ce{HNO3}$ or $ce{HClO4}$ is actually somewhat purple. We call these compounds cationic acids. So the aqua-complexes will act as acids and become deprotonated in water. For your $ce{Fe^2+}$ example it's pretty much the same. I often read in student reports that, if for example a hexaqua complex is treated with a base the $ce{OH^-}$ would exchange for one water ligand. Many then concluded that $ce{OH^-}$ had to be the stronger ligand in the spectrochemical series. This is, of course, wrong and not related to each other here. What is far more logical, at least to me is, that by coordination the water gets closer towards the highly charged metal center. But that means there is a repulsion from the $ce{H}$ in $ce{H2O}$ and the metal center since both have a positive (formal) charge. This makes it much easier to become deprotonated by a base.
But why does this stop at some points? I asked myself the same question for the example of $ce{BeO}$ reacting with $ce{OH^-}$ to form $ce{[Be(OH)4]^2-}$. Why is Beryllium oxide amphoteric forming a berrylate while $ce{MgO}$ to $ce{BaO}$ do not show this ability? It took me quite a while to find an answer to this. There is an equation by Cartledge that tries to describe this phenomenon:
$$ce{Φ = z/r}$$
Where Φ is the ionic potential, z the charge and r the radius. If $ce{Φ^{1/2}}$ is smaller than 2.2 the ion is considered basic, above 3.2 it's considered to be acidic. In the middle, it's considered amphoteric. What does that mean? It seems like there is a ratio of radius to charge. Small, highly charged ions seem to polarize the surrounding water molecules so much that they quickly lose a proton.
In your case, we are not specifically talking about oxides but I guess that something similar should be happening. The high charge on the metal and the fact that $ce{Fe}$ is already in the middle of the d-row where atomic radii decrease along the row should be enough to actually cause even more deprotonation, this time perhaps not of surrounding water molecules like in the case of $ce{BeO}$ but the ones that are already ligated to the metal center. At some point it will require additional help which happens when the pH is increased. And in fact for $ce{Fe^2+}$ a $ce{Na4[Fe(OH)6]}$ seems to exist.
I guess the comparison with the $ce{BeO}$ may perhaps be a bit far fetched but it would be an actual example where you can see how the charge to radius ratio acts on the way soluble hydroxo-complexes are formed.
$endgroup$
1
$begingroup$
Can you give a reference, other than textbook problems that often make things up, for $ce{Na4Fe(OH)6}$?
$endgroup$
– Oscar Lanzi
6 hours ago
add a comment |
$begingroup$
Since you said you have just started inorganic chemistry in school, I give you some simpler insight:
Simply, a complex metal ion has a metal ion at its center with a number of other molecules (e.g., $ce{H2O}$) or ions (e.g., $ce{CN-}$) surrounding it. When you start learning complex ions (or molecules) you must learn why complex ions of the type $ce{[M(H2O)6]^n+}$ (hexaaqua ions) are acidic. Examples are, $ce{[Fe(H2O)6]^2+}$, $ce{[Fe(H2O)6]^3+}$, $ce{[Cu(H2O)6]^2+}$, $ce{[Co(H2O)6]^2+}$, $ce{[Ni(H2O)6]^2+}$, $ce{[V(H2O)6]^2+}$, etc. The first thing you need to know is the solutions of hexaaqua ions with equal concentrations have varying range of acidity (pH of solution), which depend a lot on type of metal's charge, ionic radius, etc. See the following graph extracted from the web page, Complex Metal Ions - The Acidity of the Hexaaqua Ions.
Let's take the hexaaquairon(III), $ce{[Fe(H2O)6]^3+}$ as typical complex ion. The structure of the ion (octahedral) is given in the diagram A below and an explanation for its acidity is given in the diagram B (From Complex Metal Ions - The Acidity of the Hexaaqua Ions):
This explanation is given by visualizing the 3+ charge of the ion is located entirely on $ce{Fe}$ in the center. When one of lone pair on the $ce{O}$ of each $ce{H2O}$ form a coordinate bond with the iron, it can be visualize that these lone pairs move closer towards $ce{Fe}$. That has an effect on the electrons in all $ce{O-H}$ bonds within the complex (imagine all $ce{O}$ atoms have partial positive charges due to this electron movement). The electrons of $ce{O-H}$ bonds, in turn, get pulled towards the oxygen even more than usual because of this. That leaves each $ce{H}$ nucleus more exposed than normal (compared to that in $ce{H2O}$ molecules in the solution). The overall effect is that each of $ce{H}$ atoms is more positive than it is in ordinary water molecules. Thus, the 3+ charge is no longer located entirely on $ce{Fe}$ but spread out over the whole ion--much of it on the hydrogen atoms of coordinated water.
The $ce{H}$ atoms attached to $ce{H2O}$ ligands are sufficiently positive that they can act as Brønsted acid and can be pulled off in a acid-base reaction involving $ce{H2O}$ molecules in the solution according to the following sequence of equations illustrating the first three stages of the process:
$$ce{[Fe(H2O)6]^3+ + H2O <=> [Fe(H2O)5(OH)]^2+ + H3O+} \ce{Fe(H2O)5(OH)]^2+ + H2O <=> [Fe(H2O)4(OH)4]^+ + H3O+} \ce{Fe(H2O)4(OH)2]^+ + H2O <=> [Fe(H2O)3(OH)3] + H3O+}$$
As you see, the process end up with a complex with no charge on it after third stage. This complex is described as a Neutral Complex. Because it has no charge, thus, it doesn't dissolve in water to any extent, and will precipitate as it is formed. See below diagram for color illustration of the reaction of $ce{[NaOH}$ with $ce{[Fe(H2O)6]^2+}$ and $ce{[Fe(H2O)6]^3+}$, respectively.
$endgroup$
add a comment |
Your Answer
StackExchange.ifUsing("editor", function () {
return StackExchange.using("mathjaxEditing", function () {
StackExchange.MarkdownEditor.creationCallbacks.add(function (editor, postfix) {
StackExchange.mathjaxEditing.prepareWmdForMathJax(editor, postfix, [["$", "$"], ["\\(","\\)"]]);
});
});
}, "mathjax-editing");
StackExchange.ready(function() {
var channelOptions = {
tags: "".split(" "),
id: "431"
};
initTagRenderer("".split(" "), "".split(" "), channelOptions);
StackExchange.using("externalEditor", function() {
// Have to fire editor after snippets, if snippets enabled
if (StackExchange.settings.snippets.snippetsEnabled) {
StackExchange.using("snippets", function() {
createEditor();
});
}
else {
createEditor();
}
});
function createEditor() {
StackExchange.prepareEditor({
heartbeatType: 'answer',
autoActivateHeartbeat: false,
convertImagesToLinks: false,
noModals: true,
showLowRepImageUploadWarning: true,
reputationToPostImages: null,
bindNavPrevention: true,
postfix: "",
imageUploader: {
brandingHtml: "Powered by u003ca class="icon-imgur-white" href="https://imgur.com/"u003eu003c/au003e",
contentPolicyHtml: "User contributions licensed under u003ca href="https://creativecommons.org/licenses/by-sa/3.0/"u003ecc by-sa 3.0 with attribution requiredu003c/au003e u003ca href="https://stackoverflow.com/legal/content-policy"u003e(content policy)u003c/au003e",
allowUrls: true
},
onDemand: true,
discardSelector: ".discard-answer"
,immediatelyShowMarkdownHelp:true
});
}
});
Sign up or log in
StackExchange.ready(function () {
StackExchange.helpers.onClickDraftSave('#login-link');
});
Sign up using Google
Sign up using Facebook
Sign up using Email and Password
Post as a guest
Required, but never shown
StackExchange.ready(
function () {
StackExchange.openid.initPostLogin('.new-post-login', 'https%3a%2f%2fchemistry.stackexchange.com%2fquestions%2f109106%2fis-feoh2-soluble-in-alkalis%23new-answer', 'question_page');
}
);
Post as a guest
Required, but never shown
2 Answers
2
active
oldest
votes
2 Answers
2
active
oldest
votes
active
oldest
votes
active
oldest
votes
$begingroup$
This is a pretty interesting question. First of all, you are right, if you ever happened to see a yellow $ce{Fe^3+}$ solution that was no pure aqua-complex but more likely $ce{[Fe(H2O)5(OH)]^2-}$. The real $ce{[Fe(H2O)6]^3+}$ for example, like it is formed in conc. $ce{HNO3}$ or $ce{HClO4}$ is actually somewhat purple. We call these compounds cationic acids. So the aqua-complexes will act as acids and become deprotonated in water. For your $ce{Fe^2+}$ example it's pretty much the same. I often read in student reports that, if for example a hexaqua complex is treated with a base the $ce{OH^-}$ would exchange for one water ligand. Many then concluded that $ce{OH^-}$ had to be the stronger ligand in the spectrochemical series. This is, of course, wrong and not related to each other here. What is far more logical, at least to me is, that by coordination the water gets closer towards the highly charged metal center. But that means there is a repulsion from the $ce{H}$ in $ce{H2O}$ and the metal center since both have a positive (formal) charge. This makes it much easier to become deprotonated by a base.
But why does this stop at some points? I asked myself the same question for the example of $ce{BeO}$ reacting with $ce{OH^-}$ to form $ce{[Be(OH)4]^2-}$. Why is Beryllium oxide amphoteric forming a berrylate while $ce{MgO}$ to $ce{BaO}$ do not show this ability? It took me quite a while to find an answer to this. There is an equation by Cartledge that tries to describe this phenomenon:
$$ce{Φ = z/r}$$
Where Φ is the ionic potential, z the charge and r the radius. If $ce{Φ^{1/2}}$ is smaller than 2.2 the ion is considered basic, above 3.2 it's considered to be acidic. In the middle, it's considered amphoteric. What does that mean? It seems like there is a ratio of radius to charge. Small, highly charged ions seem to polarize the surrounding water molecules so much that they quickly lose a proton.
In your case, we are not specifically talking about oxides but I guess that something similar should be happening. The high charge on the metal and the fact that $ce{Fe}$ is already in the middle of the d-row where atomic radii decrease along the row should be enough to actually cause even more deprotonation, this time perhaps not of surrounding water molecules like in the case of $ce{BeO}$ but the ones that are already ligated to the metal center. At some point it will require additional help which happens when the pH is increased. And in fact for $ce{Fe^2+}$ a $ce{Na4[Fe(OH)6]}$ seems to exist.
I guess the comparison with the $ce{BeO}$ may perhaps be a bit far fetched but it would be an actual example where you can see how the charge to radius ratio acts on the way soluble hydroxo-complexes are formed.
$endgroup$
1
$begingroup$
Can you give a reference, other than textbook problems that often make things up, for $ce{Na4Fe(OH)6}$?
$endgroup$
– Oscar Lanzi
6 hours ago
add a comment |
$begingroup$
This is a pretty interesting question. First of all, you are right, if you ever happened to see a yellow $ce{Fe^3+}$ solution that was no pure aqua-complex but more likely $ce{[Fe(H2O)5(OH)]^2-}$. The real $ce{[Fe(H2O)6]^3+}$ for example, like it is formed in conc. $ce{HNO3}$ or $ce{HClO4}$ is actually somewhat purple. We call these compounds cationic acids. So the aqua-complexes will act as acids and become deprotonated in water. For your $ce{Fe^2+}$ example it's pretty much the same. I often read in student reports that, if for example a hexaqua complex is treated with a base the $ce{OH^-}$ would exchange for one water ligand. Many then concluded that $ce{OH^-}$ had to be the stronger ligand in the spectrochemical series. This is, of course, wrong and not related to each other here. What is far more logical, at least to me is, that by coordination the water gets closer towards the highly charged metal center. But that means there is a repulsion from the $ce{H}$ in $ce{H2O}$ and the metal center since both have a positive (formal) charge. This makes it much easier to become deprotonated by a base.
But why does this stop at some points? I asked myself the same question for the example of $ce{BeO}$ reacting with $ce{OH^-}$ to form $ce{[Be(OH)4]^2-}$. Why is Beryllium oxide amphoteric forming a berrylate while $ce{MgO}$ to $ce{BaO}$ do not show this ability? It took me quite a while to find an answer to this. There is an equation by Cartledge that tries to describe this phenomenon:
$$ce{Φ = z/r}$$
Where Φ is the ionic potential, z the charge and r the radius. If $ce{Φ^{1/2}}$ is smaller than 2.2 the ion is considered basic, above 3.2 it's considered to be acidic. In the middle, it's considered amphoteric. What does that mean? It seems like there is a ratio of radius to charge. Small, highly charged ions seem to polarize the surrounding water molecules so much that they quickly lose a proton.
In your case, we are not specifically talking about oxides but I guess that something similar should be happening. The high charge on the metal and the fact that $ce{Fe}$ is already in the middle of the d-row where atomic radii decrease along the row should be enough to actually cause even more deprotonation, this time perhaps not of surrounding water molecules like in the case of $ce{BeO}$ but the ones that are already ligated to the metal center. At some point it will require additional help which happens when the pH is increased. And in fact for $ce{Fe^2+}$ a $ce{Na4[Fe(OH)6]}$ seems to exist.
I guess the comparison with the $ce{BeO}$ may perhaps be a bit far fetched but it would be an actual example where you can see how the charge to radius ratio acts on the way soluble hydroxo-complexes are formed.
$endgroup$
1
$begingroup$
Can you give a reference, other than textbook problems that often make things up, for $ce{Na4Fe(OH)6}$?
$endgroup$
– Oscar Lanzi
6 hours ago
add a comment |
$begingroup$
This is a pretty interesting question. First of all, you are right, if you ever happened to see a yellow $ce{Fe^3+}$ solution that was no pure aqua-complex but more likely $ce{[Fe(H2O)5(OH)]^2-}$. The real $ce{[Fe(H2O)6]^3+}$ for example, like it is formed in conc. $ce{HNO3}$ or $ce{HClO4}$ is actually somewhat purple. We call these compounds cationic acids. So the aqua-complexes will act as acids and become deprotonated in water. For your $ce{Fe^2+}$ example it's pretty much the same. I often read in student reports that, if for example a hexaqua complex is treated with a base the $ce{OH^-}$ would exchange for one water ligand. Many then concluded that $ce{OH^-}$ had to be the stronger ligand in the spectrochemical series. This is, of course, wrong and not related to each other here. What is far more logical, at least to me is, that by coordination the water gets closer towards the highly charged metal center. But that means there is a repulsion from the $ce{H}$ in $ce{H2O}$ and the metal center since both have a positive (formal) charge. This makes it much easier to become deprotonated by a base.
But why does this stop at some points? I asked myself the same question for the example of $ce{BeO}$ reacting with $ce{OH^-}$ to form $ce{[Be(OH)4]^2-}$. Why is Beryllium oxide amphoteric forming a berrylate while $ce{MgO}$ to $ce{BaO}$ do not show this ability? It took me quite a while to find an answer to this. There is an equation by Cartledge that tries to describe this phenomenon:
$$ce{Φ = z/r}$$
Where Φ is the ionic potential, z the charge and r the radius. If $ce{Φ^{1/2}}$ is smaller than 2.2 the ion is considered basic, above 3.2 it's considered to be acidic. In the middle, it's considered amphoteric. What does that mean? It seems like there is a ratio of radius to charge. Small, highly charged ions seem to polarize the surrounding water molecules so much that they quickly lose a proton.
In your case, we are not specifically talking about oxides but I guess that something similar should be happening. The high charge on the metal and the fact that $ce{Fe}$ is already in the middle of the d-row where atomic radii decrease along the row should be enough to actually cause even more deprotonation, this time perhaps not of surrounding water molecules like in the case of $ce{BeO}$ but the ones that are already ligated to the metal center. At some point it will require additional help which happens when the pH is increased. And in fact for $ce{Fe^2+}$ a $ce{Na4[Fe(OH)6]}$ seems to exist.
I guess the comparison with the $ce{BeO}$ may perhaps be a bit far fetched but it would be an actual example where you can see how the charge to radius ratio acts on the way soluble hydroxo-complexes are formed.
$endgroup$
This is a pretty interesting question. First of all, you are right, if you ever happened to see a yellow $ce{Fe^3+}$ solution that was no pure aqua-complex but more likely $ce{[Fe(H2O)5(OH)]^2-}$. The real $ce{[Fe(H2O)6]^3+}$ for example, like it is formed in conc. $ce{HNO3}$ or $ce{HClO4}$ is actually somewhat purple. We call these compounds cationic acids. So the aqua-complexes will act as acids and become deprotonated in water. For your $ce{Fe^2+}$ example it's pretty much the same. I often read in student reports that, if for example a hexaqua complex is treated with a base the $ce{OH^-}$ would exchange for one water ligand. Many then concluded that $ce{OH^-}$ had to be the stronger ligand in the spectrochemical series. This is, of course, wrong and not related to each other here. What is far more logical, at least to me is, that by coordination the water gets closer towards the highly charged metal center. But that means there is a repulsion from the $ce{H}$ in $ce{H2O}$ and the metal center since both have a positive (formal) charge. This makes it much easier to become deprotonated by a base.
But why does this stop at some points? I asked myself the same question for the example of $ce{BeO}$ reacting with $ce{OH^-}$ to form $ce{[Be(OH)4]^2-}$. Why is Beryllium oxide amphoteric forming a berrylate while $ce{MgO}$ to $ce{BaO}$ do not show this ability? It took me quite a while to find an answer to this. There is an equation by Cartledge that tries to describe this phenomenon:
$$ce{Φ = z/r}$$
Where Φ is the ionic potential, z the charge and r the radius. If $ce{Φ^{1/2}}$ is smaller than 2.2 the ion is considered basic, above 3.2 it's considered to be acidic. In the middle, it's considered amphoteric. What does that mean? It seems like there is a ratio of radius to charge. Small, highly charged ions seem to polarize the surrounding water molecules so much that they quickly lose a proton.
In your case, we are not specifically talking about oxides but I guess that something similar should be happening. The high charge on the metal and the fact that $ce{Fe}$ is already in the middle of the d-row where atomic radii decrease along the row should be enough to actually cause even more deprotonation, this time perhaps not of surrounding water molecules like in the case of $ce{BeO}$ but the ones that are already ligated to the metal center. At some point it will require additional help which happens when the pH is increased. And in fact for $ce{Fe^2+}$ a $ce{Na4[Fe(OH)6]}$ seems to exist.
I guess the comparison with the $ce{BeO}$ may perhaps be a bit far fetched but it would be an actual example where you can see how the charge to radius ratio acts on the way soluble hydroxo-complexes are formed.
answered 10 hours ago
JustanotherchemistJustanotherchemist
1,814520
1,814520
1
$begingroup$
Can you give a reference, other than textbook problems that often make things up, for $ce{Na4Fe(OH)6}$?
$endgroup$
– Oscar Lanzi
6 hours ago
add a comment |
1
$begingroup$
Can you give a reference, other than textbook problems that often make things up, for $ce{Na4Fe(OH)6}$?
$endgroup$
– Oscar Lanzi
6 hours ago
1
1
$begingroup$
Can you give a reference, other than textbook problems that often make things up, for $ce{Na4Fe(OH)6}$?
$endgroup$
– Oscar Lanzi
6 hours ago
$begingroup$
Can you give a reference, other than textbook problems that often make things up, for $ce{Na4Fe(OH)6}$?
$endgroup$
– Oscar Lanzi
6 hours ago
add a comment |
$begingroup$
Since you said you have just started inorganic chemistry in school, I give you some simpler insight:
Simply, a complex metal ion has a metal ion at its center with a number of other molecules (e.g., $ce{H2O}$) or ions (e.g., $ce{CN-}$) surrounding it. When you start learning complex ions (or molecules) you must learn why complex ions of the type $ce{[M(H2O)6]^n+}$ (hexaaqua ions) are acidic. Examples are, $ce{[Fe(H2O)6]^2+}$, $ce{[Fe(H2O)6]^3+}$, $ce{[Cu(H2O)6]^2+}$, $ce{[Co(H2O)6]^2+}$, $ce{[Ni(H2O)6]^2+}$, $ce{[V(H2O)6]^2+}$, etc. The first thing you need to know is the solutions of hexaaqua ions with equal concentrations have varying range of acidity (pH of solution), which depend a lot on type of metal's charge, ionic radius, etc. See the following graph extracted from the web page, Complex Metal Ions - The Acidity of the Hexaaqua Ions.
Let's take the hexaaquairon(III), $ce{[Fe(H2O)6]^3+}$ as typical complex ion. The structure of the ion (octahedral) is given in the diagram A below and an explanation for its acidity is given in the diagram B (From Complex Metal Ions - The Acidity of the Hexaaqua Ions):
This explanation is given by visualizing the 3+ charge of the ion is located entirely on $ce{Fe}$ in the center. When one of lone pair on the $ce{O}$ of each $ce{H2O}$ form a coordinate bond with the iron, it can be visualize that these lone pairs move closer towards $ce{Fe}$. That has an effect on the electrons in all $ce{O-H}$ bonds within the complex (imagine all $ce{O}$ atoms have partial positive charges due to this electron movement). The electrons of $ce{O-H}$ bonds, in turn, get pulled towards the oxygen even more than usual because of this. That leaves each $ce{H}$ nucleus more exposed than normal (compared to that in $ce{H2O}$ molecules in the solution). The overall effect is that each of $ce{H}$ atoms is more positive than it is in ordinary water molecules. Thus, the 3+ charge is no longer located entirely on $ce{Fe}$ but spread out over the whole ion--much of it on the hydrogen atoms of coordinated water.
The $ce{H}$ atoms attached to $ce{H2O}$ ligands are sufficiently positive that they can act as Brønsted acid and can be pulled off in a acid-base reaction involving $ce{H2O}$ molecules in the solution according to the following sequence of equations illustrating the first three stages of the process:
$$ce{[Fe(H2O)6]^3+ + H2O <=> [Fe(H2O)5(OH)]^2+ + H3O+} \ce{Fe(H2O)5(OH)]^2+ + H2O <=> [Fe(H2O)4(OH)4]^+ + H3O+} \ce{Fe(H2O)4(OH)2]^+ + H2O <=> [Fe(H2O)3(OH)3] + H3O+}$$
As you see, the process end up with a complex with no charge on it after third stage. This complex is described as a Neutral Complex. Because it has no charge, thus, it doesn't dissolve in water to any extent, and will precipitate as it is formed. See below diagram for color illustration of the reaction of $ce{[NaOH}$ with $ce{[Fe(H2O)6]^2+}$ and $ce{[Fe(H2O)6]^3+}$, respectively.
$endgroup$
add a comment |
$begingroup$
Since you said you have just started inorganic chemistry in school, I give you some simpler insight:
Simply, a complex metal ion has a metal ion at its center with a number of other molecules (e.g., $ce{H2O}$) or ions (e.g., $ce{CN-}$) surrounding it. When you start learning complex ions (or molecules) you must learn why complex ions of the type $ce{[M(H2O)6]^n+}$ (hexaaqua ions) are acidic. Examples are, $ce{[Fe(H2O)6]^2+}$, $ce{[Fe(H2O)6]^3+}$, $ce{[Cu(H2O)6]^2+}$, $ce{[Co(H2O)6]^2+}$, $ce{[Ni(H2O)6]^2+}$, $ce{[V(H2O)6]^2+}$, etc. The first thing you need to know is the solutions of hexaaqua ions with equal concentrations have varying range of acidity (pH of solution), which depend a lot on type of metal's charge, ionic radius, etc. See the following graph extracted from the web page, Complex Metal Ions - The Acidity of the Hexaaqua Ions.
Let's take the hexaaquairon(III), $ce{[Fe(H2O)6]^3+}$ as typical complex ion. The structure of the ion (octahedral) is given in the diagram A below and an explanation for its acidity is given in the diagram B (From Complex Metal Ions - The Acidity of the Hexaaqua Ions):
This explanation is given by visualizing the 3+ charge of the ion is located entirely on $ce{Fe}$ in the center. When one of lone pair on the $ce{O}$ of each $ce{H2O}$ form a coordinate bond with the iron, it can be visualize that these lone pairs move closer towards $ce{Fe}$. That has an effect on the electrons in all $ce{O-H}$ bonds within the complex (imagine all $ce{O}$ atoms have partial positive charges due to this electron movement). The electrons of $ce{O-H}$ bonds, in turn, get pulled towards the oxygen even more than usual because of this. That leaves each $ce{H}$ nucleus more exposed than normal (compared to that in $ce{H2O}$ molecules in the solution). The overall effect is that each of $ce{H}$ atoms is more positive than it is in ordinary water molecules. Thus, the 3+ charge is no longer located entirely on $ce{Fe}$ but spread out over the whole ion--much of it on the hydrogen atoms of coordinated water.
The $ce{H}$ atoms attached to $ce{H2O}$ ligands are sufficiently positive that they can act as Brønsted acid and can be pulled off in a acid-base reaction involving $ce{H2O}$ molecules in the solution according to the following sequence of equations illustrating the first three stages of the process:
$$ce{[Fe(H2O)6]^3+ + H2O <=> [Fe(H2O)5(OH)]^2+ + H3O+} \ce{Fe(H2O)5(OH)]^2+ + H2O <=> [Fe(H2O)4(OH)4]^+ + H3O+} \ce{Fe(H2O)4(OH)2]^+ + H2O <=> [Fe(H2O)3(OH)3] + H3O+}$$
As you see, the process end up with a complex with no charge on it after third stage. This complex is described as a Neutral Complex. Because it has no charge, thus, it doesn't dissolve in water to any extent, and will precipitate as it is formed. See below diagram for color illustration of the reaction of $ce{[NaOH}$ with $ce{[Fe(H2O)6]^2+}$ and $ce{[Fe(H2O)6]^3+}$, respectively.
$endgroup$
add a comment |
$begingroup$
Since you said you have just started inorganic chemistry in school, I give you some simpler insight:
Simply, a complex metal ion has a metal ion at its center with a number of other molecules (e.g., $ce{H2O}$) or ions (e.g., $ce{CN-}$) surrounding it. When you start learning complex ions (or molecules) you must learn why complex ions of the type $ce{[M(H2O)6]^n+}$ (hexaaqua ions) are acidic. Examples are, $ce{[Fe(H2O)6]^2+}$, $ce{[Fe(H2O)6]^3+}$, $ce{[Cu(H2O)6]^2+}$, $ce{[Co(H2O)6]^2+}$, $ce{[Ni(H2O)6]^2+}$, $ce{[V(H2O)6]^2+}$, etc. The first thing you need to know is the solutions of hexaaqua ions with equal concentrations have varying range of acidity (pH of solution), which depend a lot on type of metal's charge, ionic radius, etc. See the following graph extracted from the web page, Complex Metal Ions - The Acidity of the Hexaaqua Ions.
Let's take the hexaaquairon(III), $ce{[Fe(H2O)6]^3+}$ as typical complex ion. The structure of the ion (octahedral) is given in the diagram A below and an explanation for its acidity is given in the diagram B (From Complex Metal Ions - The Acidity of the Hexaaqua Ions):
This explanation is given by visualizing the 3+ charge of the ion is located entirely on $ce{Fe}$ in the center. When one of lone pair on the $ce{O}$ of each $ce{H2O}$ form a coordinate bond with the iron, it can be visualize that these lone pairs move closer towards $ce{Fe}$. That has an effect on the electrons in all $ce{O-H}$ bonds within the complex (imagine all $ce{O}$ atoms have partial positive charges due to this electron movement). The electrons of $ce{O-H}$ bonds, in turn, get pulled towards the oxygen even more than usual because of this. That leaves each $ce{H}$ nucleus more exposed than normal (compared to that in $ce{H2O}$ molecules in the solution). The overall effect is that each of $ce{H}$ atoms is more positive than it is in ordinary water molecules. Thus, the 3+ charge is no longer located entirely on $ce{Fe}$ but spread out over the whole ion--much of it on the hydrogen atoms of coordinated water.
The $ce{H}$ atoms attached to $ce{H2O}$ ligands are sufficiently positive that they can act as Brønsted acid and can be pulled off in a acid-base reaction involving $ce{H2O}$ molecules in the solution according to the following sequence of equations illustrating the first three stages of the process:
$$ce{[Fe(H2O)6]^3+ + H2O <=> [Fe(H2O)5(OH)]^2+ + H3O+} \ce{Fe(H2O)5(OH)]^2+ + H2O <=> [Fe(H2O)4(OH)4]^+ + H3O+} \ce{Fe(H2O)4(OH)2]^+ + H2O <=> [Fe(H2O)3(OH)3] + H3O+}$$
As you see, the process end up with a complex with no charge on it after third stage. This complex is described as a Neutral Complex. Because it has no charge, thus, it doesn't dissolve in water to any extent, and will precipitate as it is formed. See below diagram for color illustration of the reaction of $ce{[NaOH}$ with $ce{[Fe(H2O)6]^2+}$ and $ce{[Fe(H2O)6]^3+}$, respectively.
$endgroup$
Since you said you have just started inorganic chemistry in school, I give you some simpler insight:
Simply, a complex metal ion has a metal ion at its center with a number of other molecules (e.g., $ce{H2O}$) or ions (e.g., $ce{CN-}$) surrounding it. When you start learning complex ions (or molecules) you must learn why complex ions of the type $ce{[M(H2O)6]^n+}$ (hexaaqua ions) are acidic. Examples are, $ce{[Fe(H2O)6]^2+}$, $ce{[Fe(H2O)6]^3+}$, $ce{[Cu(H2O)6]^2+}$, $ce{[Co(H2O)6]^2+}$, $ce{[Ni(H2O)6]^2+}$, $ce{[V(H2O)6]^2+}$, etc. The first thing you need to know is the solutions of hexaaqua ions with equal concentrations have varying range of acidity (pH of solution), which depend a lot on type of metal's charge, ionic radius, etc. See the following graph extracted from the web page, Complex Metal Ions - The Acidity of the Hexaaqua Ions.
Let's take the hexaaquairon(III), $ce{[Fe(H2O)6]^3+}$ as typical complex ion. The structure of the ion (octahedral) is given in the diagram A below and an explanation for its acidity is given in the diagram B (From Complex Metal Ions - The Acidity of the Hexaaqua Ions):
This explanation is given by visualizing the 3+ charge of the ion is located entirely on $ce{Fe}$ in the center. When one of lone pair on the $ce{O}$ of each $ce{H2O}$ form a coordinate bond with the iron, it can be visualize that these lone pairs move closer towards $ce{Fe}$. That has an effect on the electrons in all $ce{O-H}$ bonds within the complex (imagine all $ce{O}$ atoms have partial positive charges due to this electron movement). The electrons of $ce{O-H}$ bonds, in turn, get pulled towards the oxygen even more than usual because of this. That leaves each $ce{H}$ nucleus more exposed than normal (compared to that in $ce{H2O}$ molecules in the solution). The overall effect is that each of $ce{H}$ atoms is more positive than it is in ordinary water molecules. Thus, the 3+ charge is no longer located entirely on $ce{Fe}$ but spread out over the whole ion--much of it on the hydrogen atoms of coordinated water.
The $ce{H}$ atoms attached to $ce{H2O}$ ligands are sufficiently positive that they can act as Brønsted acid and can be pulled off in a acid-base reaction involving $ce{H2O}$ molecules in the solution according to the following sequence of equations illustrating the first three stages of the process:
$$ce{[Fe(H2O)6]^3+ + H2O <=> [Fe(H2O)5(OH)]^2+ + H3O+} \ce{Fe(H2O)5(OH)]^2+ + H2O <=> [Fe(H2O)4(OH)4]^+ + H3O+} \ce{Fe(H2O)4(OH)2]^+ + H2O <=> [Fe(H2O)3(OH)3] + H3O+}$$
As you see, the process end up with a complex with no charge on it after third stage. This complex is described as a Neutral Complex. Because it has no charge, thus, it doesn't dissolve in water to any extent, and will precipitate as it is formed. See below diagram for color illustration of the reaction of $ce{[NaOH}$ with $ce{[Fe(H2O)6]^2+}$ and $ce{[Fe(H2O)6]^3+}$, respectively.
answered 7 hours ago


Mathew MahindaratneMathew Mahindaratne
6149
6149
add a comment |
add a comment |
Thanks for contributing an answer to Chemistry Stack Exchange!
- Please be sure to answer the question. Provide details and share your research!
But avoid …
- Asking for help, clarification, or responding to other answers.
- Making statements based on opinion; back them up with references or personal experience.
Use MathJax to format equations. MathJax reference.
To learn more, see our tips on writing great answers.
Sign up or log in
StackExchange.ready(function () {
StackExchange.helpers.onClickDraftSave('#login-link');
});
Sign up using Google
Sign up using Facebook
Sign up using Email and Password
Post as a guest
Required, but never shown
StackExchange.ready(
function () {
StackExchange.openid.initPostLogin('.new-post-login', 'https%3a%2f%2fchemistry.stackexchange.com%2fquestions%2f109106%2fis-feoh2-soluble-in-alkalis%23new-answer', 'question_page');
}
);
Post as a guest
Required, but never shown
Sign up or log in
StackExchange.ready(function () {
StackExchange.helpers.onClickDraftSave('#login-link');
});
Sign up using Google
Sign up using Facebook
Sign up using Email and Password
Post as a guest
Required, but never shown
Sign up or log in
StackExchange.ready(function () {
StackExchange.helpers.onClickDraftSave('#login-link');
});
Sign up using Google
Sign up using Facebook
Sign up using Email and Password
Post as a guest
Required, but never shown
Sign up or log in
StackExchange.ready(function () {
StackExchange.helpers.onClickDraftSave('#login-link');
});
Sign up using Google
Sign up using Facebook
Sign up using Email and Password
Sign up using Google
Sign up using Facebook
Sign up using Email and Password
Post as a guest
Required, but never shown
Required, but never shown
Required, but never shown
Required, but never shown
Required, but never shown
Required, but never shown
Required, but never shown
Required, but never shown
Required, but never shown
t0II,PL8x0nX5o7VkHmuQHU 6j6C9vhFI,lEIO PnjFV5vq1qfUcI09Rk 69Xwb4 sl9c
$begingroup$
Actually precipitate isn't [Fe(OH)2(H2O)4]. Precipitation starts with it, but it's rather complex condensation/polymerisation process.
$endgroup$
– Mithoron
9 hours ago